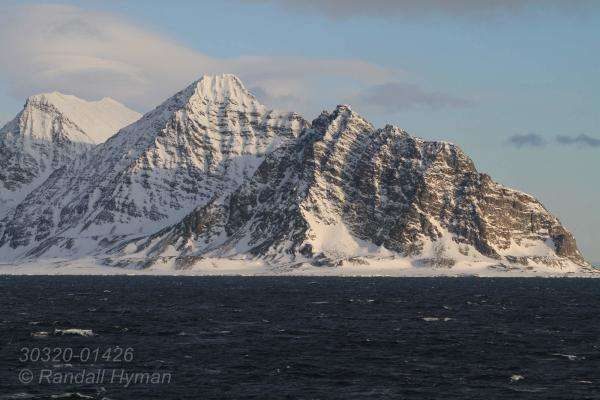
Consigned to my bunk all night by gut-wrenching seas, I wistfully thought back to terra firma on mainland Norway a few days earlier. Before boarding the University of Tromsø’s research ship, R/V Helmer Hanssen, I had watched a team of engineers put finishing touches on two golf-cart-sized crafts brimming with scientific instruments. Now the so-called landers were lashed to our aft deck looking like NASA probes bound for planetary exploration, except these were headed for our own planet’s continental shelf in the Svalbard archipelago, Norway’s northernmost territory, 800 miles from the North Pole.
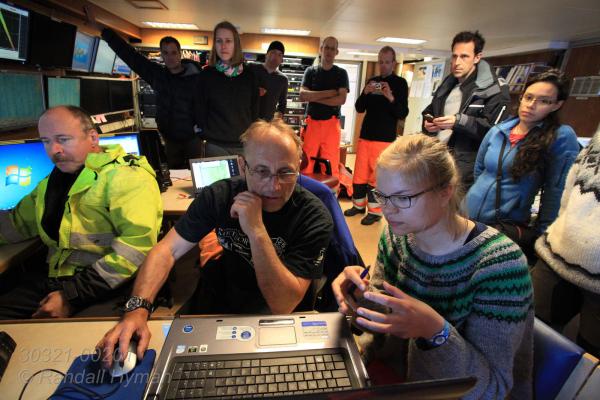
The seafloor landers would be monitoring chemistry, particularly methane seeping from the seabed, for an entire year to glean a better understanding of how that substance might affect Earth’s future climate. Methane, the chief component of the natural gas used in homes and industry, is 25 times more potent than carbon dioxide as a greenhouse gas. At nearly half a million dollars each, the new landers represented the single most ambitious effort by Norway’s CAGE institute (Center for Arctic Gas Hydrate, Environment and Climate) to advance seafloor methane research.
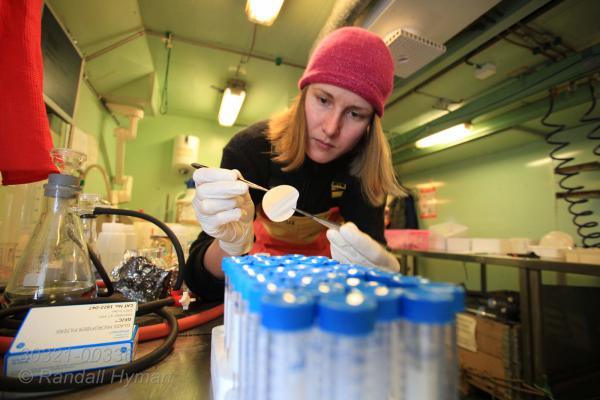
CAGE scientists already have evidence that oceans can absorb some of the methane that naturally bubbles up from the seafloor, but knowing how much is too much could be crucial to predicting climate change. Scientists want answers fast since a much bigger threat than methane is its cousin, an ice-like substance called methane hydrate.
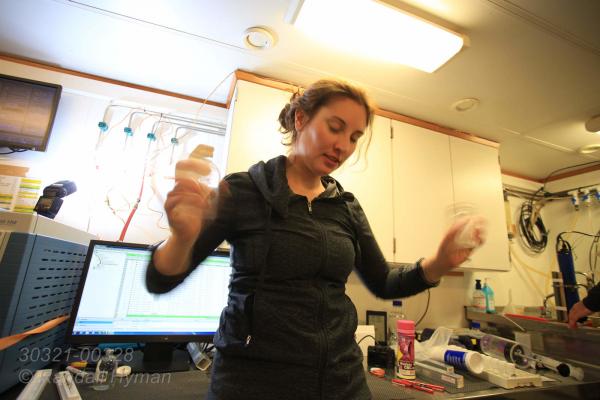
Trapped semi-frozen beneath seafloors worldwide, methane hydrate is a sleeping giant that can expand 160 times when changed to gas. That’s exciting news for oil companies, but terrifying for climate scientists. Although some hydrates exist on land beneath permafrost, 99% of known reserves are buried beneath the seafloor. Some of Norway’s largest reservoirs lie right along the continental shelf where a slight rise in temperature could thaw and unleash a methane hydrate monster affecting more than just climate.
“Methane hydrates serve as a kind of cement of the sediments,” Dr. Peter Linke told me the next morning. As a scientist at Germany’s GEOMAR Helmholtz Centre for Ocean Research, Linke works closely with CAGE and was on our cruise to supervise deployment of the German-built landers. “Our fear is that if this cement gets dissolved, sediment might slide down the slope. If a large volume of sediment is moving, this might cause a tsunami, which has happened before.”
Promise and peril
Where climatologists see great peril, oil companies see great promise. While its economy is based largely on oil, Norway is looking at all other possibilities, especially since global reserves of hydrate-bound methane have been estimated as high as 10,000 gigatonnes– twice the world’s total petroleum, natural gas and coal reserves combined.
Norway is not alone in putting a lot of effort and money into researching these seemingly innocuous bubbles at the bottom of the ocean. In recent years, methane has become the subject of intense international scrutiny, both as an environmental threat and as an energy windfall. The Japanese have shown it is possible to extract methane from seafloor hydrates, but an uncontrolled, quick increase of methane from a meltdown could produce a vicious cycle: the more methane emitted into the atmosphere, the more the oceans warm and the more hydrates continue to destabilize.
Like oil and coal, natural gas forms deep beneath the seafloor under great pressure and heat where ancient organic matter decomposes without oxygen. Bacteria also produce such gas through anaerobic decomposition, similar to bogs and swamps on land. Both geothermal and bacterial methane emanate from the seafloor in features called seeps, but gaseous seeps are just a small part of the story. On the way up through the seabed where cold temperatures and pressure often converge in the perfect combination, lots of methane gets trapped as solid hydrates.
Research on seeps and hydrates began in the 1980s, around the time scientists noticed natural carbonate chimneys displayed as curios in the yards of fishermen in Corvallis, Oregon. Trawlers had accidentally dredged the chimneys from the seafloor, but this serendipitous discovery helped launch a new field of science. Like many budding disciplines, research on marine methane attracted scientists of all kinds, from biologists to geochemists to geologists to physicists.
“We were all trying to construct explanations for the who, what and why,” Linke told me. A few years later, he and his boss, one of the first to stumble across the fishermen’s curios, took a crude methane sniffer aboard a German research vessel in the Barents Sea. Right away they found seeps everywhere, but their good luck ran out when a massive storm hit.
“We thought we’d never make it back,” Linke recalled. “It was a surprise October gale. For three days we had these huge waves and the boat swinging back and forth at a 42-degree tilt. It was crazy. We had heavy icing and smashed windows and labs filling with water.”
His description made my “gut-wrenching seas” look like a splash in the kiddie pool, but whatever weather was in store, the week-long schedule promised to be grueling. The team of 16 scientists would be sampling water and air within a 400-square-kilometer sector known for methane seeps as well as launching the two landers. Adjacent to our survey site, under the seabed at the very edge of the continental shelf, sat large hydrate reservoirs. Should those hydrates ever begin bubbling off, CAGE research could be crucial in predicting the consequences.
In a methane hydrate, the gas molecule is actually trapped in a “cage” of water molecules, hence the name of Norway’s CAGE institute. Hydrates look and feel like ice, but are not a frozen gas. In fact, they are more akin to minerals because they have a geometric architecture rather than a random structure like true ice. Another distinction: unlike frozen water, a lump of methane hydrate can be set on fire.
Hydrates require low temperatures and high pressure to remain intact. There are normally plenty of both in the Arctic Ocean– lots of cold and tons of pressure beneath the sea floor. Along Svalbard’s continental shelf, however, hydrates reside in relatively shallow waters near the top of the seabed where required pressure is marginal such that even a small change in water temperature can initiate a meltdown.
Plumbing methane’s secrets
It’s not all bad news, though. According to Dr. John Pohlman, a US Geological Survey scientist working with CAGE, methane seeps might actually help reduce greenhouse gas.
“I call it the Seep Fertilization Hypothesis,” Pohlman explained as he set up the cruise’s main experiment. “On this same survey last year I noticed that where methane content was highest at the surface, there was also a depletion of carbon dioxide in the water. We don’t yet understand why, but the release of methane bubbles from the seafloor seems to stimulate uptake of CO2 from the water, which leads to uptake of CO2 from the overlying atmosphere.”
In Pohlman’s model, the water above methane seeps is like a garden full of algae and methane-loving bacteria called methanotrophs. As methane bubbles upward, it carries nutrient-rich deep water with it that fertilizes algae at the surface. Like all plants, algae consume carbon dioxide and produce oxygen. The concept of methane seeps as sponges of greenhouse gases is new for oceanographers.
To duplicate the previous year’s observations, Pohlman and two colleagues were busy below deck rigging three machines to measure methane and carbon dioxide, as collected outside the ship through funnels and tubing. Two more experiments were crucial: test the activity of methanotrophs and determine what happens to excess carbon dioxide, which can create carbonic acid and acidify seawater. Dying coral reefs around the world are evidence that even slight acidification can be devastating.
Biochemist Dr. Helge Niemann of the University of Basel in Switzerland was preparing to measure methanotrophs in a lab above Pohlman’s. The afternoon we arrived off the coast of Svalbard, I found him mixing radioactive cocktails.
“Think of it like a dinner table and our guests are methanotrophs,” Niemann explained as his assistant carefully worked under a chemical hood. “We want to know how hungry they are, how many they are, and who they are.”
To measure hunger, Niemann and his assistant were preparing vials of radioactive hydrogen called tritium to add into water samples throughout the week. When active, methanotrophs produce carbon dioxide and water. The amount of radioactive water produced in water samples would reveal how much they ate.
To count how many guests showed up, he would be using a tagging process involving fluorescent probes that light up DNA sequences in the bacteria for actual counting under a fluorescence microscope. And to produce a true guest list, he would be taking samples back to his lab in Switzerland for a cloning procedure that amplifies tiny bits of DNA thousands of times so that individual species can be readily identified. Another colleague was sampling seawater acidity and dissolved carbon content at different depths to measure the impact of excess carbon dioxide. All was ready, but the first order of business was to deploy of one of the landers.
Seafloor landing
Linke and others were already on deck in hard hats and life vests gently maneuvering the 1.6-ton unit with a cargo crane. After a year of preparation, the pyramidal lander dangled above cobalt seas, attached to a detachable launch module equipped with a video camera. The roster of high-tech devices aboard the lander read like a Christmas toy list for oceanographers, with half a ton of lithium batteries included to run the package for a full year. In addition to three separate sensors for measuring methane, carbon dioxide and pH (acidity), the lander also carried and a sonar to monitor gas bubbles, a fluorescence meter to detect algal blooms and a Doppler instrument to track ocean currents.
In minutes, the team lowered the lander into the sea. As the cable began winding out, Linke ditched his safety gear and rushed inside to the instrument room to flip on the video feed from the lander’s detachable launch frame. Amid an array of computer screens, a grainy blue scene appeared as the craft descended 240 meters (787 feet) toward the seafloor.
Scientists and engineers anxiously clustered in the darkened room to watch, with only quiet murmurs and the throb of the ship’s engines filling the silence. It felt like an Apollo moon landing. Suddenly the seafloor came into view and the cable was brought to a full stop. As the ship slowly edged toward the predesignated landing site, occasional rocks appeared and a shark sashayed by.
Then the moment of truth: bubbles. As expected, methane seeps were plentiful here, but the challenge was to position the lander among them, not on top of them, where optimal observations could be made. CAGE’s cruise leader sat nervously beside Linke. When the critical moment arrived, he gave her the honor of releasing the lander. With a click of the computer keyboard, the video image careened wildly as remotely-fired explosives shot the launcher off its payload and allowed the lander to set down.
Applause and handshakes filled the room, but the celebration was brief. The lander would be retrieved a year later for data download and analysis. For now, the team turned its attention to several days of water sampling using a cluster of canisters fixed to a circular steel carousel called a rosette. At each measuring station on our map, the crew lowered the rosette to just above the seafloor and then began reeling it back in, snapping open ports on successive canisters to fill them at specific depths.
Over the next 96 hours, with a short pause for launching the second lander, scientists gathered around the rosette every hour after it was pulled aboard at 65 separate stations,, draining canisters into dozens of glass vials for each experiment. They worked continuously in rotating shifts, catching what sleep they could.
By the end of the week, exhausted and bleary-eyed, Pohlman had documented several clear examples of carbon dioxide levels mysteriously dropping above methane seeps, just as he had one year earlier. Whether methane seeps prove to be greenhouse-gas sponges or not, the bigger issue remains their cousins, methane hydrates. If warmer seas cause hydrates to begin breaking down and releasing gas, what then? Toward the end of the cruise, Linke mentioned new research addressing this very concern.
Sweet news
“GEOMAR has a project we call SUGAR because, like CAGE’s name, sugar resembles the structure of the gas hydrate molecule,” Linke told me. “The idea is to substitute the methane in the hydrate with carbon dioxide in order to harvest methane as energy, and on the other hand, get rid of carbon dioxide, which is a threat as well.”
Not only does the SUGAR process sequester harmful carbon dioxide and reap methane for energy, it also leaves behind a compound which is more stable and heat tolerant than methane hydrate. GEOMAR’s patented process is scientific sleight-of-hand performed under specific pressure and temperature, magically swapping carbon dioxide for methane in its tiny hydrate “cage.” The project is now being field tested in the Black Sea where methane hydrates occur in large quantities. If SUGAR works there, hydrates could sweeten life for nations worldwide.
On our last day, the team treated itself to a bit of tourism in the Russian ghost town of Pyramiden, nestled deep in a fjord. It was a perfect conclusion to our trip. Pyramiden is an abandoned coal mining village and a reminder that, although there is plenty of coal, gas and oil in the Arctic, harvesting any of it is difficult.
Founded by Swedes in 1910 and sold to the Soviet Union in 1927, Pyramiden once boasted a population of over 1000 and was heavily subsidized as a model communist village. As a 1924 signatory of the Svalbard Treaty, which recognizes Norway’s sovereignty but allows treaty members to engage in commercial activity there, the Soviets maintained Pyramiden for much of the 20th century until the empire’s fall. Dwindling profits and a devastating plane crash that killed 141 miners, with families and crew, in 1996 led to the last residents leaving in 1998.
Walking from the dock past mounds of coal and scrap metal, we came to a monolithic town sign with a small rail car parked beneath identifying the contents as the last ton of coal mined at Pyramiden. In the town’s heyday, no extravagance had been spared to lure miners from their meager lives in Russia and Ukraine to this remote Soviet paradise. Frost-resistant grasses imported from Ukraine stretched along broad boulevards lined with modern brick buildings.
An expansive basketball gymnasium, natatorium and movie theater graced the sports complex, but dresses, musical instruments and other sundries lay about in various rooms as if everyone had suddenly vanished. In the ghostly main square, a lonely statue of Lenin stared toward the massive, ancient glacier that presided over Pyramiden’s meteoric rise and fall, underlining humanity’s fleeting presence in this harsh land of ice, storm and darkness.
As Arctic coal and oil lose their glow and petroleum giants like Royal Dutch Shell walk away from billions of dollars of investment, natural gas grows ever more attractive. It is a cleaner fossil fuel increasingly used in power plants, home heating and vehicles. If extracting methane hydrates on an industrial scale becomes viable, many nations will have access to substantial reserves.
At the Arctic Frontiers conference in Tromsø last January, Norwegian Prime Minister Erna Solberg declared that gas would play a crucial role in oil-rich Norway’s transition from fossil fuels to renewables. With the Arctic warming twice as fast as the global average and pollution from coal and oil a major factor, Norwegian research on methane hydrates is very much on the front burner.
Sidebar: Fueling misery
Less than 20 years after Russians abandoned Svalbard’s ill-fated Pyramiden coal mine, Norwegians are packing their own bags in the nearby coal-mining town of Longyearbyen. With a population of nearly 2200, it is one of only two large settlements left in Svalbard, the other being the Russian-owned coal town of Barentsburg. Years of rock-bottom coal prices have recently forced the Store Norske Spitsbergen mining company to announce the layoff of 150 of its 250 employees and the suspension of most operations with the help of a $59 million government loan buyout. The shutdown will severely impact Longyearbyen’s economy.
Meanwhile, an announcement from the University of Washington in October has fueled fears among oceanographers worldwide that warming seas could unleash large amounts of greenhouse gas if semi-frozen methane hydrates “boil off.” UW scientists published a study showing that methane bubble plumes off the Northwest Pacific coast are emanating from depths where methane hydrates occur and where anomalous warm currents from Siberia may be the culprit.
“It is unusual to see plumes at this critical depth [of 500 meters],” says oceanographer H. Paul Johnson, one of the study’s lead authors, “and we have previously confirmed warming at that same depth of .3 degrees Celsius.”
Methane hydrates are common along continental margins in places like the Northwest Pacific and Svalbard. Although hydrates are a potential new energy source, warmer oceans may “harvest” them before humans do, possibly accelerating climate change and increasing ocean acidification while triggering submarine landslides and tsunamis.